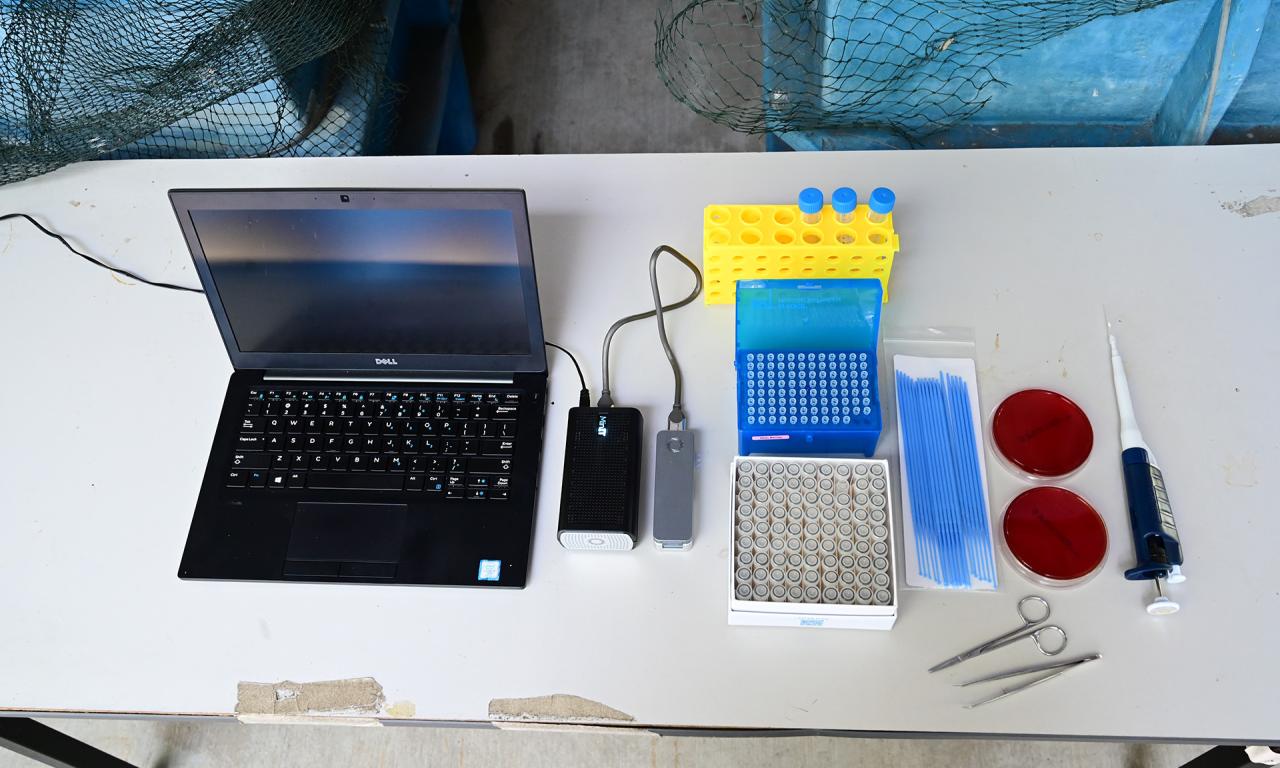
A team of researchers from Malaysia, Australia, New Zealand, Thailand, and the UK is harnessing the power of genome sequencing to help Aquaculture farmers manage fish diseases. Until recently, this was not possible as the technology was not only costly but the genome sequencing process required time and coordination between different specialist labs. But this changed with the invention of MinION from Oxford Nanopore Technologies, a small and easy to use USB device that makes the genomic sequencing cheaper and faster.
The Rapid Genomics Sequencing team is now developing a cloud-based database to get high-resolution information from raw nanopore sequences allowing them to track outbreaks in close to real-time. The information they generate will then be accessed in a user-friendly format via a smartphone, enabling aquaculture inspectors to provide biosecurity advice and management plans to farmers in the palm of their hands. It will also inform local manufacturers what to put into custom vaccines and which farms need it. With real-time data accurately identifying what is killing the fish, local universities and start-ups will be able to produce simple vaccines for aquaculture health practitioners to vaccinate new stocks of fish before dissemination onto farms.
The team getting ready to collect samples from fish for disease diagnostic investigation in the premises of the fish farm.
The team getting ready to collect samples from fish for disease diagnostic investigation in the premises of the fish farm.
In a demonstration at the WorldFish office, the team created a mock-up scenario of how this portable Lab-in-a-Backpack system works. If a fish farmer experiences a disease outbreak on his farm, local aquaculture inspectors collect samples from diseased fish and send them to a nearby lab where trained operators will be able to process them for whole-genome sequencing, with identification and typing of a bacterial pathogen taking just a few hours.
“Current diagnosis-by-sequencing workflows employ a range of different methods for DNA extraction, sequencing and analysis, and many labs tend to curate their own reference sequence databases in house. This makes it difficult to compare results between laboratories and limits our ability to interpret infection dynamics in a timely manner. Our team is developing a simple and reliable surveillance workflow in which pathogen sequence data is generated on the MinION device and identified in real-time using an intuitive web application. Behind this interface sits a powerful learning algorithm and gold-standard reference sequence database that is continually updated as emergent pathogens are identified.” - said Dr Shaun Wilkinson, of Wilderlab New Zealand.
Tracing of pathogens by molecular epidemiology is extremely powerful. It reveals the fine variations between strains of a particular group of bacteria which helps biosecurity officials to trace the origin of infection and warn farmers or breeders of any problems before moving animals further between regions or countries. For example, a bug’s DNA might show that the strain is new to the fish in country A, but caused an outbreak in a nearby country B a few months earlier.
“Rapid genome sequencing provides all of the information we need to make informed decisions on disease control without the need for pathogen-specific tests and tools that might be very expensive,” said Dr Andrew Barnes of The University of Queensland (UQ), who is part of the team behind the WorldFish-led proposal. “The sequences enable you to infer the origin of a pathogen; see how it is evolving and moving through different environments and across international borders. Most importantly, it allows you to identify possible antimicrobial resistance genes and virulence factors relevant to vaccine formulation. With this kind of knowledge, we can provide very specific advice on how to control and prevent disease outbreaks”.
loading a rapid barcoded samples onto a flongle flow cell
The research team is sharing and demonstrating their portable Lab-in-a-backpack system with other researchers in Asia and Africa to make rapid genomic sequencing of aquaculture pathogens gradually available. Ultimately, this innovation will enable fish farmers and aquaculture enterprises to track and deal with fish disease in real-time, and thus minimize potential loss of food, incomes and sustainable business.
“We believe this technology will have an incredible impact on the aquaculture industry globally in terms of providing safe, affordable and nutritious fish, especially in developing countries, said Dr Jerome Delamare-Deboutteville, aquaculture scientist with WorldFish.
By gathering information on the latest outbreaks as they happen, the system should pick up valuable epidemiological data, trends in drug resistance or the rise of new bugs that are resistant to current antibiotics. If everything goes well we will have this technology available for aquaculture and fish health experts as well as the data for policymakers to better manage and respond to fish disease outbreaks.”
Explaining the science behind the "Lab-in-a-backpack":
- Big Data: is a term that describes the large volume of data – both structured and unstructured (ref). The CGIAR Platform for Big Data in Agriculture is where information becomes power: the power to predict, prescribe, and produce more food, more sustainably. It democratizes decades of agricultural data empowering analysts, statisticians, programmers and more to mine information for trends and quirks, and develop rapid, accurate and compelling recommendations for farmers, researchers, and policymakers (ref).
- Artificial Intelligence (AI): makes it possible for machines to learn from experience, adjust to new inputs and perform human-like tasks. Most AI examples that you hear about today – from chess-playing computers to self-driving cars – rely heavily on deep learning and natural language processing. Using these technologies, computers can be trained to accomplish specific tasks by processing large amounts of data and recognizing patterns in the data (ref).
- Machine learning: is a method of data analysis that automates analytical model building. It is a branch of artificial intelligence based on the idea that systems can learn from data, identify patterns and make decisions with minimal human intervention (ref).
- Learning algorithm: at its most basic, machine learning uses programmed algorithms that receive and analyze input data to predict output values within an acceptable range. As new data is fed to these algorithms, they learn and optimize their operations to improve performance, developing ‘intelligence’ over time. There are four types of machine learning algorithms: supervised, semi-supervised, unsupervised and reinforcement (ref).
- Base Pair (BP): is two chemical bases bonded to one another forming a "rung of the DNA ladder." The DNA molecule consists of two strands that wind around each other like a twisted ladder. Each strand has a backbone made of alternating sugar (deoxyribose) and phosphate groups. Attached to each sugar is one of four bases--adenine (A), cytosine (C), guanine (G), or thymine (T). The two strands are held together by hydrogen bonds between the bases, with adenine forming a base pair with thymine, and cytosine forming a base pair with guanine (ref).
- Next-Generation Sequencing (NGS): is the catch-all term used to describe a number of different modern sequencing technologies that have revolutionized the study of genomics and molecular biology. NGS is a high-throughput sequencing method used to determine a portion of the nucleotide sequence of an organism’s genome. NGS is capable of processing multiple DNA and RNA sequences in parallel rapidly (ref).
- Illumina sequencing: in NGS, vast numbers of short reads are sequenced in a single stroke. To do this, firstly the input DNA sample must be cleaved into short sections. The length of these sections will depend on the particular sequencing machinery used. In Illumina sequencing, 100-150 base pair (bp) reads are used. Somewhat longer fragments are ligated to generic adaptors and annealed to a slide using the adaptors. PCR is carried out to amplify each read, creating a spot with many copies of the same read. They are then separated into single strands to be sequenced (ref).
- Nanopore sequencing: is a unique, scalable technology that enables direct, real-time analysis of long DNA or RNA fragments. It works by monitoring changes to electrical current as nucleic acids are passed through a protein nanopore. The resulting signal is decoded to provide the specific DNA or RNA sequence (ref).
- Whole-genome sequencing: is the process of determining the complete DNA sequence of an organism's genome (e.g. human, cow, fish, bacterium, virus). By combining two datasets from Illumina (short reads) and Nanopore (long reads), we are now able to rapidly close the complete genome of any bacterial pathogen affecting fish and other animals.
- Bioinformatics: is an interdisciplinary field of science that combines biology, computer science, information engineering, mathematics and statistics to analyze and interpret the biological data (ref). Bioinformatics predominantly focus on three types of large datasets available in molecular biology: macromolecular structures, genome sequences, and the results of functional genomics experiments (e.g. expression data). Bioinformatics employs a wide range of computational techniques including sequence and structural alignment, database design and data mining, macromolecular geometry, phylogenetic tree construction, prediction of protein structure and function, gene finding, and expression data clustering (ref).
- Reference sequence database: is a comprehensive, integrated, non-redundant, well-annotated set of reference sequences including genomic, transcript, and protein (ref).
- Minion USB sequencer: is the only portable, real-time device for DNA and RNA sequencing of ultra-long read lengths (hundreds of kilobase). A kilobase (kb) is a unit of measurement in molecular biology equal to 1000 base pairs of DNA or RNA. The Minion weighs under 100 g and plugs into a PC or laptop using high-speed USD 3.0 cable or used alongside the MinIT device for real-time, powerful analyses. Not constrained to a laboratory environment, it has been used up a mountain, in a jungle, in the arctic and on the International Space Station (ref).
- MinIT: is a companion to the MinION personal DNA/RNA sequencer eliminating the need for a dedicated laptop for nanopore sequencing with MinION. It is pre-configured with the software that controls the MinION (MinKNOW), carries out data acquisition and performs base-calling. As a self-contained unit, MinIT has a small footprint, 290 g only, with Bluetooth and Wi-Fi enabled allowing users to control their experiments in remote locations or in laboratories using a laptop, tablet or smartphone (ref).
- Antimicrobial resistance (AMR): the ability of microbes to resist the effects of medicines and other chemicals that are used to control them, presents a formidable threat to health and sustainable development. Antimicrobial treatments and medicines are used within human health and in terrestrial and aquatic food production to manage infection and sometimes to improve farm production. Resistance can emerge in people, in animals, and in the environment, and may spread between people and animals. AMR is a ‘one health’ issue wherein the health of people, animals, and the environment are interlinked. The development of resistance in one location and in one setting can affect the health of people and animals more broadly. Likewise, efforts to combat resistance require efforts in all areas. The aquatic environment is recognized as a key site for the emergence and transmission of AMR (ref).
- Autogenous vaccine: is prepared from a specific pathogen (e.g. a bacterium or virus) isolated from diseased animals (e.g. fish) at a particular farm experiencing a disease outbreak. After inactivation, the autogenous vaccine is then used to immunize/vaccinate new stock on any farms that have the same pathogen type thereby securing the output of the next crop, preventing further spread of the same organism between farms and removing the need for antibiotics.
Acknowledgments
This research project was undertaken in the framework of the CGIAR Research Program on Fish Agri-Food Systems (FISH) led by WorldFish in collaboration with the University of Queensland and Wilderlab. This project received additional funding by the CGIAR Antimicrobial Resistance Hub on AMR related activities and from the CGIAR Platform for Big Data in Agriculture as part of the 2019 Inspire Challenge prize.
The data in the database were provided as part of two Australian Research Council (ARC) funded projects: Discovery Project DP120102755 Fighting Disease on Farms and Linkage Project LP130100242: A Pan-genome reverse vaccinology approach to streptococcal vaccination in fish. Some bacterial DNA samples and viral amplicon products for sequencing were kindly provided by researchers from Centex/Mahidol University/BIOTEC. Viral PCR amplicon sequences were analysed by a researcher from Kasetsart University. Researchers from the University of Exeter and the Centre for Environment Fisheries and Aquaculture Science (Cefas) provided their support on analyses of viral sequences extracted from cell cultures.
Related Publications:
- Rapid genomic detection of aquaculture pathogens
- A first look at the Oxford Nanopore MinION sequencer
- Aphid: an R package for analysis with profile hidden Markov models
- Microevolution of Streptococcus agalactiae ST-261 from Australia indicates dissemination via imported tilapia and ongoing adaptation to marine hosts or environment
- Whole-genome analysis of Yersinia ruckeri isolated over 27 years in Australia and New Zealand reveals geographical endemism over multiple lineages and recent evolution under host selection
- Production without medicalisation
- The AMR problem: demanding economies, biological margins, and co-producing alternative strategies
- Why antimicrobial resistance in aquaculture systems matters for the One Health approach
- Raising awareness of antimicrobial resistance in rural aquaculture practice in Bangladesh through digital communications: a pilot study